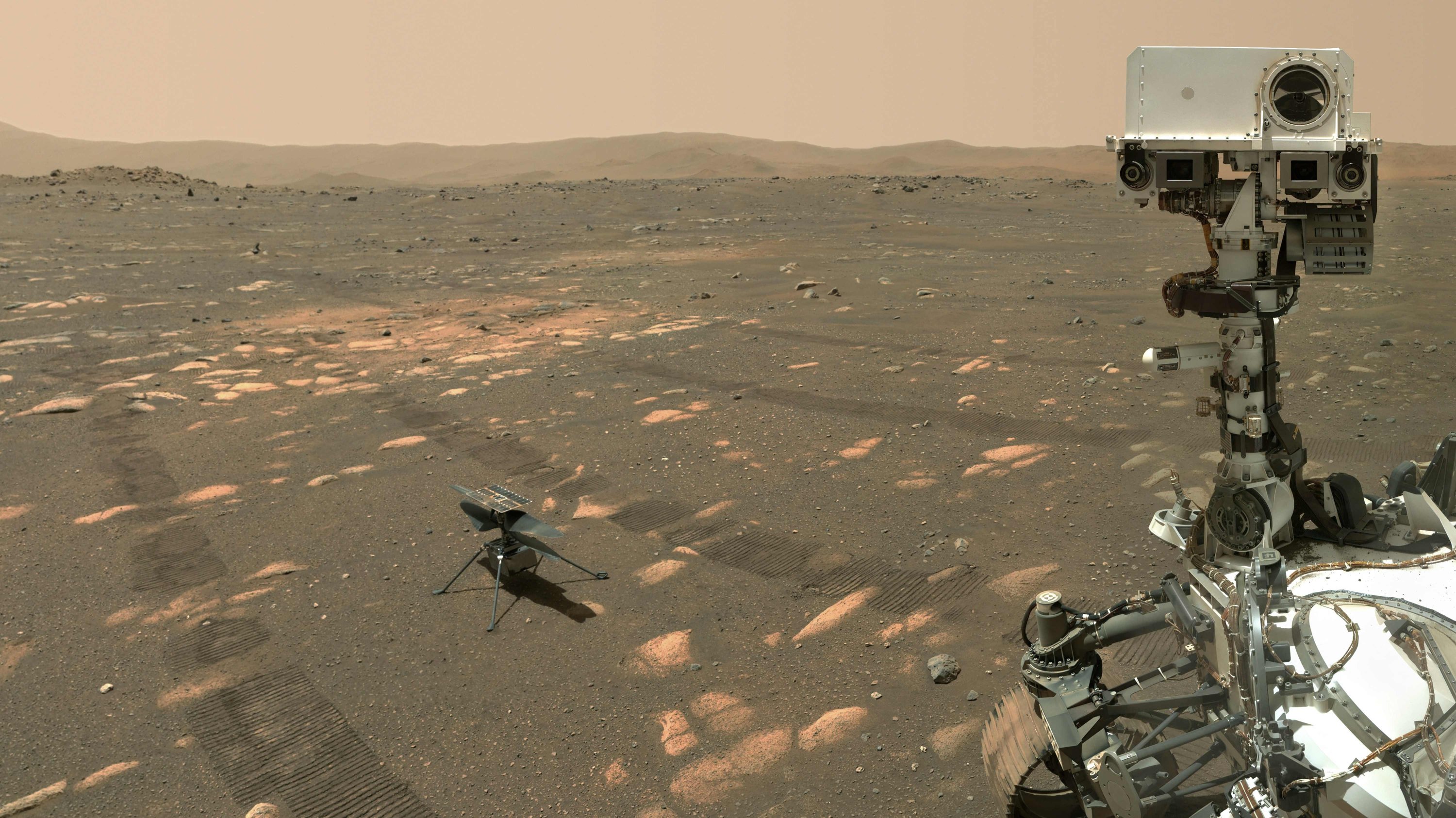
1,000 Martian sols ago, two further ambassadors from Earth arrived on the Red Planet, winched safely down onto the floor of Jezero Crater by a hovering “skycrane”. Since then, both have performed their work near-flawlessly over a period of almost 3 terrestrial years – one doing do for far, far longer than its designers and operators had ever hoped. They are, of course, the Mars 2020 mission rover Perseverance and its companion “Mars Helicopter” Ingenuity.
The mission actually arrived on Mars on February 18th 2021, but the passing of 1,000 sols (as the local Martian day is called) is an excellent opportunity to review the Mars 2020 mission as a whole, and look to the future.
Ingenuity had a planned mission duration of 90 terrestrial days during which it was expected to be able to make up to five flights; no-one really knew how well the craft’s batteries, electronics and mechanical systems would stand up to the hostile conditions on Mars once operations got underway. But as of December 2nd, 2023, the 1.8 kg drone has complete 64 flight and clocked up just over 2 hours of airborne time. In doing so, it has proven that entirely automated flight on other planets without direct human control is possible, and that a small, camera-equipped aerial vehicle can work in tandem with ground units to help reconnoitre potential routes of exploration and identify potential points of scientific interest.
Perseverance, meanwhile, has spent the intervening time studying an ancient river delta within the crater, believed to have formed as water poured down from the plains above early in Mars’ history, depositing clays and other minerals as they gradually flowed outwards and eventually gave rise to a lake within Jezero. The primary mission for the rover has thus far been to explore the delta and seek both evidence of past habitability and search for actual biosignatures indicative of past life. In doing so, Perseverance has gathered 23 air and soil samples, some of which may be returned to Earth in a future (if controversial, in terms of NASA funding) sample-return mission.
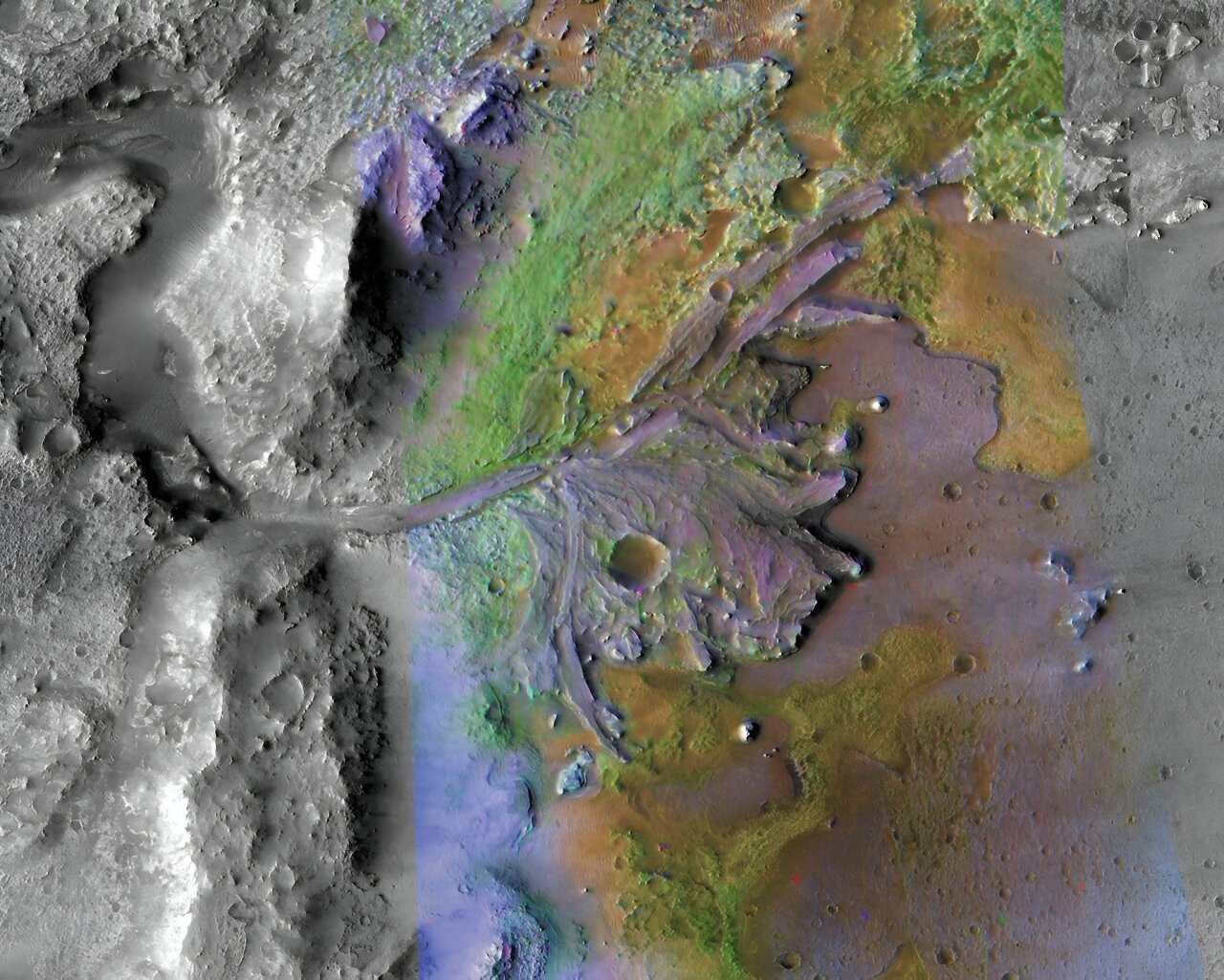
The data gathered by the rover confirms that Jezero Crater – originally formed some 4 billion years ago via an asteroid impact – was subject to multiple periods of flooding which took place over an extended period commencing several hundred million years after the crater was formed. These periods of flooding initially gave rise to the deposition of sandstone and mudstone in the crater, suggesting a modest lake was created. Later, this lake underwent a more sustained period of cyclic flooding and evaporation, giving rise to the deposition of salt-rich mudstones as the waters expanded and contracted.
At its peak, it is believed the lake was perhaps 35 kilometres in diameter and 30 metres deep. Later, as Mars’ climate became more erratic, the crater was subjected to sudden, violent bursts of flooding from above, with large rocks and boulders from outside of the crater being deposited within it by repeated flash floods before the lake – and all surface water on Mars – slowly vanished, being lost to space through evaporation as the atmosphere was lost, or ras a result of it retreating underground, where it froze.
Of the samples gathered and studies by the rover’s on-board science lab, many carried tantalising markers which might be associated with the formation of basic forms of life. These include carbonates, minerals that form in watery environments often favourable to the development of organic molecules (although the molecules themselves could be the result of either organic or inorganic reactions within the water). The rover has also found quantities of fine-grained silica and deposits of phosphate, both of which have been rich in carbonates, and which are respectively known to both preserve fossilised microbes and help microbes kick-start their life processes here on Earth – although evidence of them doing the same on Mars remains elusive. Some of the carbonate-carrying phosphates have been found to contain iron, something again associated with life here on Earth.
December 2023 is a key month for Perseverance, as it brings to a close the rover’s fourth science campaign within Jezero Crater and the start of a new endeavour. Commencing in 2024, Perseverance will follow the course of the river bed back towards the crater wall – a distance of around 4 km – to where mission personnel believe they have located an “easy” climb up the crater walls and which intersects the river’s channel at its lower end.
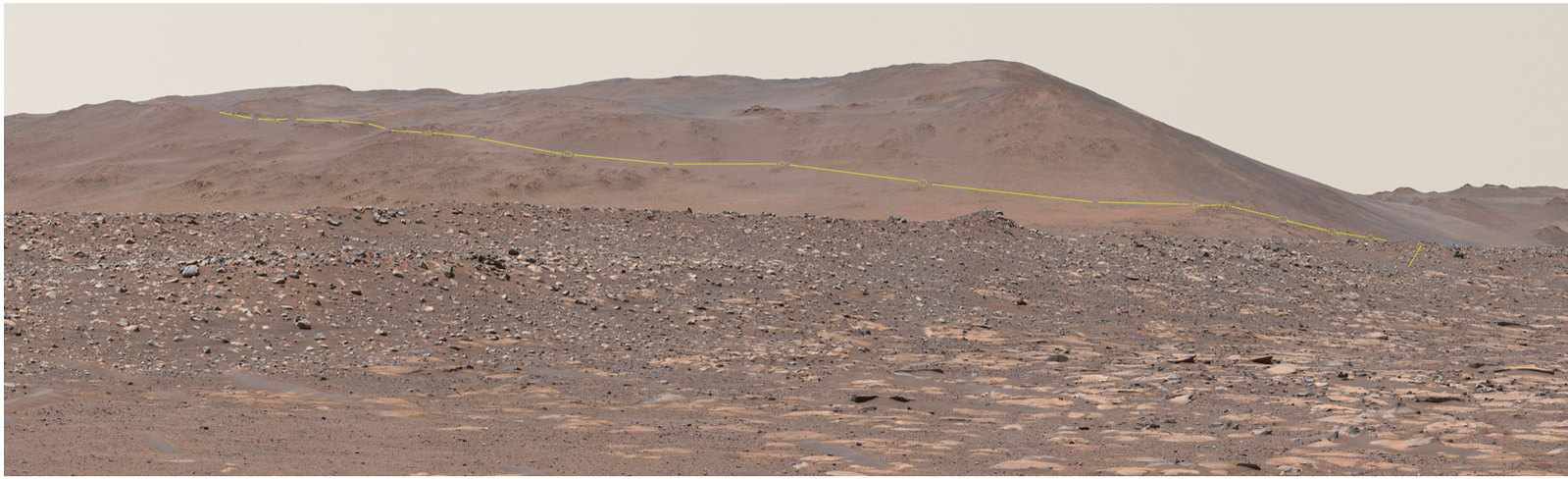
Climbing the crater up to the plains above will expose Perseverance’s science instruments to bedrock and material even older then the outflow plain it has thus far studied, allowing it to reach back to the time the crater was formed. Along the way it will be able to both study the changing rocks and any atmospheric changes as it climbs upwards. As well as analysing the rock samples it gathers, the rover will also store some in the remaining 13 sample tubes contained in its belly, allowing them to be cached together with some of the remaining tubes of material gathered from the crater floor so that an alternate collection of samples can await the arrival of the still-to-be-fully-defined sample return mission, should landing within Jezero itself prove too difficult for the proposed lander part of the mission, and the samples cached there are abandoned.
Video Promotes Rosalind Franklin
If fortune favours the unfortunate, the next rover to trundle across the surface of Mars will be Europe’s long-awaited Rosalind Franklin. Originally called the ExoMars rover, this vehicle has suffered a number of setbacks during its 20 years in development and pre-flight hell. However, (and touching large amounts of wood, given I have something of a loose association with the mission), things are currently on course for an October 2028 launch, that the European Space Agency felt confident enough to release a new promotional video showcasing the mission.
Some 60% heavier and slightly larger than NASA’s Mars Exploration Rovers Opportunity and Spirit, the European rover is, like them, solar-powered. It also shares a similar mission arc as both of the MER rovers and the nuclear-powered Curiosity and Perseverance: to locate evidence for water on Mars and seek out evidence for past signs of life. However, in one respect its mission does differ, as Rosalind Franklin will also focus seeking evidence for current microbial life on Mars.
To assist with the latter, the rover will be equipped with a drilling mechanism capable of reaching up to two metres beneath the planet’s surface – far beyond depths so far plumbed in the search for evidence of Martian microbial life – with the samples gathered then put through extensive study and analysis by the rover’s multiple science systems.
The landing site for the mission is Oxia Planum, a region located between two outflow channel systems: Mawrth Vallis to the northeast and Ares Vallis to the southwest. Scientists believe this region will contain remnants of the planet’s wetter past, increasing the potential for finding evidence for past or even current microbial life on the planet. Once there – the flight to Mars will take almost exactly 2 years, courtesy of the capabilities of its launch vehicle – Rosalind Franklin will travel up to 70 metres a day when on the move, with an overall primary mission expected to last some 7 months.
Voyager 1 Hits Problems
Humanity’s first interstellar ambassador, Voyager 1, is now just over 47 years into its voyage and more than 162 AU (or 24 billion kilometres) from Earth – and like all of us as we grow older, it is increasingly showing signs of its age. Already, the more energy-intensive science instruments on the lonely spacecraft have been shut down, and engineers have had to repeatedly work their way gingerly around assorted problems the craft has encountered; such is the distance separating vehicle and home planet that even the tiniest errors risks breaking all communications.

Most recently, Voyager 1 has started having issues with two key systems: the Flight Data System (FDS) and the Telemetry Modulation Unit (TMU). The latter is responsible for transmitting to Earth data on the spacecraft’s condition, orientation, etc., together with information from its operational science instruments, and receiving and managing communications from Earth. The data it sends is gathered by the three computers of the FDS, which combine everything obtained from the other instruments and sub-systems into a single package for the TMU to send. Except recently, all the TMU has been sending is a repeating pattern of meaningless binary, although it has continued to act on messages from Earth.
It had been thought the problem lies with the TMU itself, but after careful and painfully slow diagnoses (round-trip communications between Voyager 1 and Earth are on the order of 45 hours); the problem was found to be within the FDS. Over the weekend of December 9th/10th, mission engineers ordered the FDS to perform a sequential restart, which it was hoped would kick-start the system into once again passing meaningful data to the TMU. It didn’t.
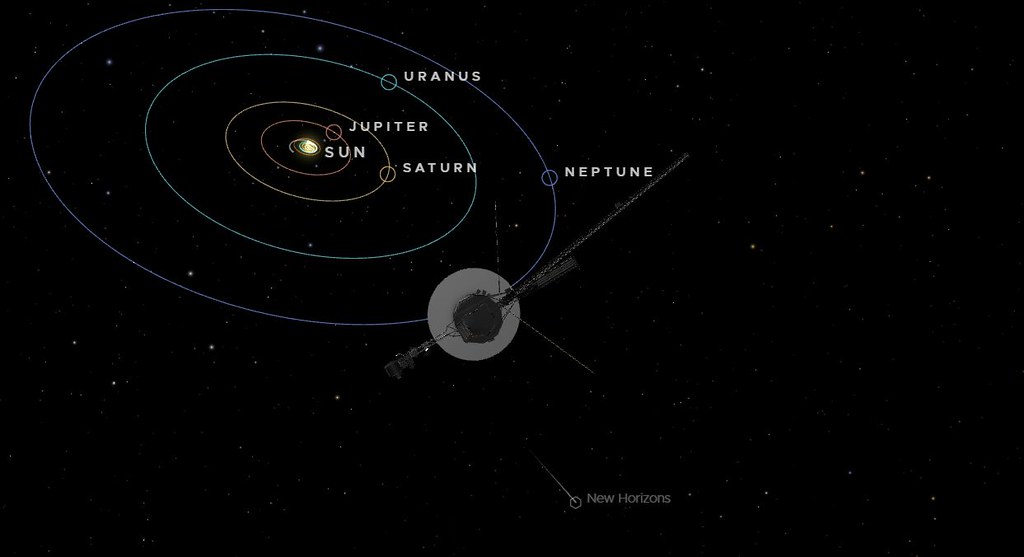
So currently, Voyager 1 remains capable of receiving commands from Earth, but it cannot provide any understandable feedback on whether anything succeeded, or what systems are trying to report back through the FDS. As such, the Voyager mission team have indicated it will take several weeks to formulate a new plan of action in order to try to resolve the problem.
Spaceplanes, Spaceplanes
Both the United States and China were due to launch their highly secretive, automated “spaceplanes” this past week – although as it turned out, only one of them actually did so.
The United States X-37B programme had been due to commence its seventh mission – and the fourth flight of the 2nd of the two X-37B craft the US Space Force and US Air Force jointly operate – on December 14th. It was to be the first flight of the craft atop a SpaceX Falcon Heavy, seen as offering the craft the ability to fly missions at much higher orbits than can be achieved using its over launch vehicles – the ULA Atlas V 501and the Falcon 9 Block 4 -, potentially allowing for more flexible and even longer-duration on-orbit operations.
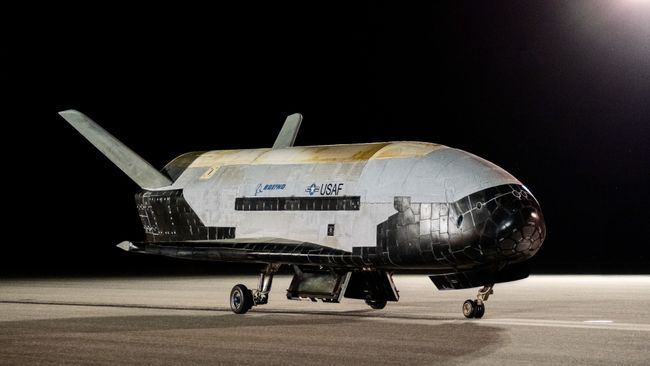
The cause of the delay has not been stated, but appears to have been called by SpaceX rather than the US DoD, and following the postponement, the Falcon Heavy was removed from Pad 39A at Kennedy Space Centre. At the time of writing, no revised launch target has been announced.
China, however, so no such delays in the third flight of its Shenlong “Divine Dragon” spaceplane, which lifted-off from the Jiuquan Satellite Launch Centre on December 14th, as planned, using a Long March 2F booster.
Little is actually known about the Chinese vehicle – although there is an emerging consensus that it is potentially similar in overall size and form to the US X-37B. The craft first flew the craft in September 2020 and then was launched a second time in August 2022 – this mission lasting for 276 days, which is still a small fraction of the time the US craft tends to spend in orbit (908 days on its last mission). That said, the second Shenlong mission did cause surprise and concern in the west when it apparently launch / placed / jettisoned something into space – China has remained tight-lipped as to what it was.
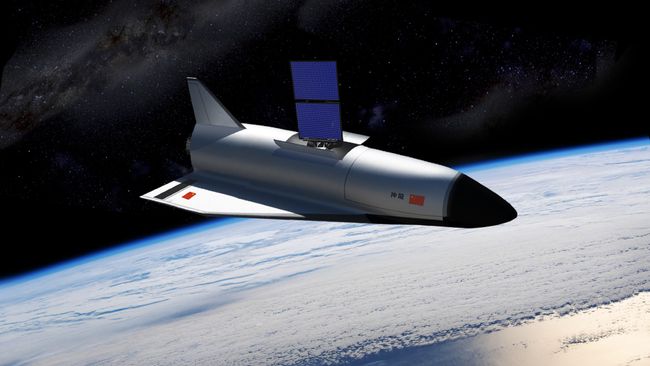
No information on the flight or its potential duration has been given by the Chinese authorities, with the official statement post-launch something of a laconic repetition of the announcements which followed the first two flights of the vehicle.
The test spacecraft will be in orbit for a period of time before returning to the domestic scheduled landing site. During this period, it will carry out reusable technology verification as planned to provide technical support for the peaceful use of space.
– Official and bland Chinese statement following the latest Shenlong launch
That both vehicles were originally intended to launch so close together is not a coincidence. The USSC/USAF has been very open in its desire to learn more about the Chinese vehicle’s purpose and capabilities – and the China probably likewise want to know more about the American vehicle. Thus, having them in space at the same time allows the two nations to observe one another’s craft via Earth-based means and – perhaps – mimic the manoeuvrings of one another’s vehicles.